Local Tsunami Warning
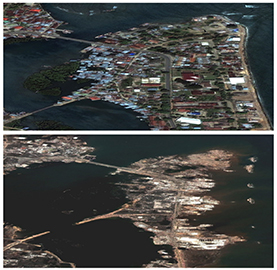
Local tsunamis impact coastal communities onshore of subduction zones leaving little time for issuing tsunami warnings. The first waves of local tsunamis can reach the coastline within 10-15 minutes (e.g., in Puget Sound, Cascadia) to 30-60 minutes (e.g., in Japan). In comparison with ocean basin-wide tsunami warning systems, which are well advanced and may have hours of lead time, local tsunami warning systems are still being developed. Two examples from a long list of catastrophic earthquakes and tsunamis in recent history point to the importance of local tsunami warning. The December 26, 2004 Mw9.3 Sumatra-Andaman earthquake and tsunami resulted in 250,000 casualties, the majority of them on the nearby island of Sumatra, Indonesia with tsunami inundation heights of up to 30 m (Paris et al. 2007). The March 11, 2011 Mw9.0 Tohoku-oki earthquake generated a tsunami with inundationheights as high as 40 m resulting in over 18,000 casualties, with extensive and long-term damage to infrastructure, in particular the Fukushima nuclear facility, and the economic collapse of the near-source coastline (Mimura et al. 2011; Mori, Takahashi, and Group 2012; Yun and Hamada 2014; Hayashi 2012). To put this into perspective, damage and death from a great earthquake on the Cascadia megathrust and subsequent tsunami, the last one was in 1700 (Satake et al. 1996; Atwater et al. 1995), is likely to be comparable to that of the 2011 Mw9.0 Tohoku-oki event.
The key input for mitigating the damage from a local tsunami is the rapid estimate of earthquake magnitude and determination of the rupture mechanism, since most seismogenic earthquakes occur on thrust faults (Figure). Current seismic methods saturate for large magnitude earthquakes (link to seismogeodesy). Thus, they are limited to observing distant (teleseismic) waves and direct measurements of tsunami waves by deep-ocean buoys and tide gauges that are suitable for basin-wide warnings, but not timely enough for local tsunamis. Local GNSS networks do not have these limitations. GNSS receivers do not clip and GNSS-derived 3-D station displacements do not exhibit magnitude saturation. However, they are not accurate enough to detect first-arrival seismic P-waves. Seismogeodesy provides a broadband seismic instrument that also provides real-time static, as well as dynamic displacements and seismic velocities, which is able to detect P-waves.
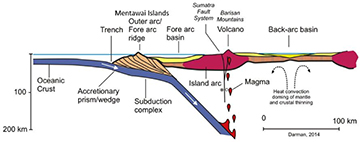
Tsunami warning in the U.S. is the responsibility of the National Oceanic and Atmospheric Administration through its Tsunami Warning Centers– the National Tsunami Warning Center (NTWC) in Alaska and the Pacific Tsunami Warning Center (PTWC) in Hawaii. SOPAC is part of an ongoing NASA-funded and overseen collaborative effort to develop a local tsunami warning system for the TWCs, include Central Washington University, the Jet Propulsion Laboratory, Univ. Washington and Univ. Oregon. The system is dubbed GWORM (GPS in Earthworm) and builds upon the existing Earthworm seismic systems at the TWCs. GWORM consists of a series of processes to ingest GNSS displacement data, as well as seismogeodetic displacements and velocities, and plug-in modules that allow for a hierarchy of real- to near real-time products. These include automatic P-wave picking and hypocenter estimation (Goldberg and Bock, 2017), magnitude scaling relationships based on peak ground displacement (PGD) (Crowell et al., 2013, Melgar et al., 2015; Goldberg et al., 2018), finite-source CMT solutions (Melgar et al., 2013) and static fault slip models as input for rapid tsunami warning and modeling (Melgar and Bock, 2015). The architecture of SOPAC’s system and its relationship to GWORM is shown below.
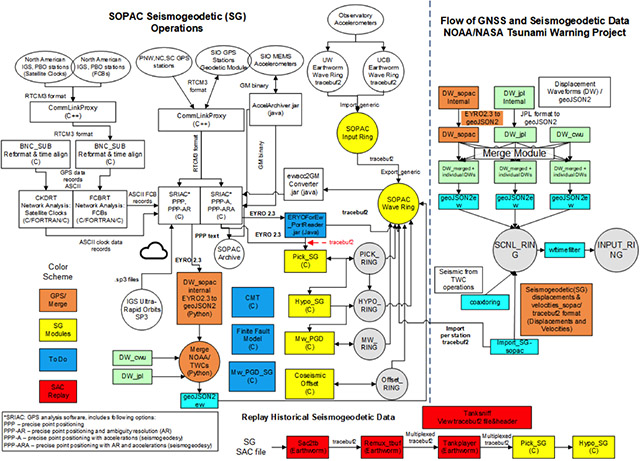
Local Tsunami Modeling
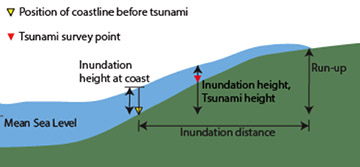
NOAA’s TWCs’ systems are focused on estimating earthquake magnitude and fault mechanism to provide general levels of evacuation warnings that may be updated as time proceeds. GNSS plays an important role here. The timeliness of GNSS-based tsunami warnings available from these rapid methods is important in issuing a general evacuation warning for local populations. However, rigorous modeling of a local tsunami in near-real time is a complex operation, mainly because of significant cpu requirements. Nevertheless, more refined methods can provide additional and more accurate information on tsunami propagation and arrival times, geographical extent, inundation distance and height, and run-up. This information can also improve the resolution of fault slip models (Melgar and Bock, 2015). The approach is based on inversion of some combination of land-based geodetic and seismic data to first estimate a staticor kinematicfault slip model from which sea-floor upliftis inferred. Next, seafloor uplift combined with detailed topography and bathymetric maps can then be used as input to detailed tsunami warningfor coastal and islands adjacent to the earthquake rupture zone.
SOPAC has made contributions in local tsunami modeling. We showed that land-based GNSS data may allow for an improved tsunami model (Melgar and Bock 2013). However, the resolving power of land-based data to shallow fault slip responsible for large uplift and tsunamis can be quite limited. Incorporating direct data from offshore GNSS buoys (kinematic displacements), ocean-bottom pressure sensors and tide gauges, as they become available, can provide increased fault slip resolution and model accuracy, but with increased latency (Melgar and Bock, 2015) (Tsunami modeling video); in many cases it can still provide sufficient time to issue a refined tsunami prediction. A study of the resolution of the different data sets used to model the 2011 Tohoku-oki event shows that GNSS displacement time series are most sensitive to slip closest to the coast and almost completely insensitive to slip close to the trench, while seismogeodetic velocities are sensitive to slip anywhere on the fault. The tsunami wave observations are most sensitive to slip near the trench. Each data set, used independently provides limited resolving power, while their combination affords a substantial improvement (Melgar and Bock 2015).
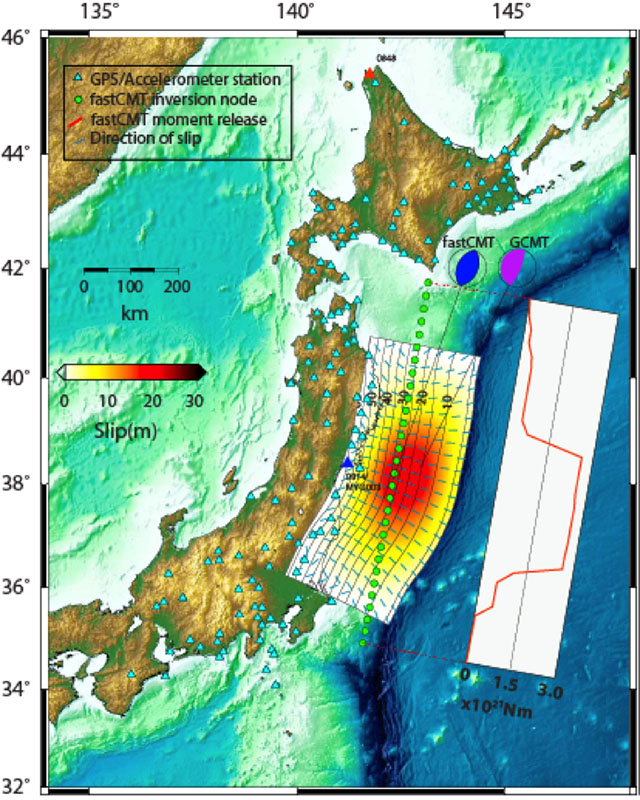
Seafloor positioning by five ocean-surface GPS buoys and sea-bottom acoustic transponders using the method of GPS-Acoustic (GPS-A) pioneered by Fred Spiess at SIO, and fortuitously installed off the Tohoku coast between 2000 and 2004 above the 2011 hypocenter showed seafloor displacements up to 24 m in the horizontal and 3 m of vertical uplift (Sato et al. 2011). An acoustic transponder within 50 m of the trench recorded 31±1 m of horizontal motion (Kido et al. 2011). An important addition to local tsunami warning is an extensive network of seafloor cables installed in Japan to transfer data in real time to the Japan Meteorological Agency. Two submarine networks for transmitting seafloor data have recently been established in the region of the 2011 Tohoku-oki event (Mochizuki et al. 2016).
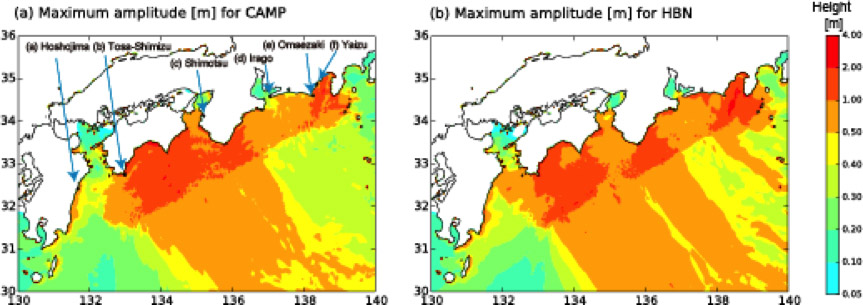
SOPAC recently published a paper with Shun-Ichi Watanabe, a visiting scientist from the Japan Coast Guard, on tsunami scenarios at the Nankai Trough (Watanabe et al., 2018), a major tectonic feature in southwestern Japan off theKii Peninsula and Shikoku Island, using both land-based and sea floor velocities. These data enable us to resolve the spatial distribution of the slip deficit rate between earthquakes. Considering a tectonic block model and the transient postseismic deformation due to the major earthquakes in the region, the slip deficit rate between the two relevant blocks can be estimated. In our study, we corrected for the time-dependent postseismic deformation of the 2004 southeastern off the Kii Peninsula earthquakes ( 7.1, 7.4), which had led to the underestimation of the slip deficit rate in earlier studies. We modeled the post-earthquake viscoelastic relaxation using the 3D Finite Element Model with bi-viscous Burgers rheology, as well as the afterslip on the finite faults. The corrected GPS-A and land-based GNSS data were aligned to the existing tectonic model and used to estimate the slip deficit rate on the plate boundary, for which two different plate interface geometries are tested. We then calculated the coseismic displacements and tsunami wave propagation assuming that a hundred years of constant slip deficit accumulation were released instantaneously. Although two tsunami models indicate roughly similar results, the peak height of the tsunami wave and its arrival time at the several points depend on the plate interface geometry. The similarities and the differences specify a range of possible local tsunami scenarios constrained by the geodetically-derived slip deficit models.
SOPAC References
- Watanabe, S., Y. Bock, D. Melgar, and K. Tadokoro (2018), Tsunami scenarios based on interseismic models along the Nankai Trough, Japan from seafloor and onshore geodesy, J. Geophys. Res. Solid Earth, in press.
- Bock, Y. and D. Melgar (2016), Physical Applications of GPS Geodesy: A Review, Rep. Prog. Phys. 79, 10, doi:10.1088/0034-4885/79/10/106801.
- Melgar, D., R. M. Allen, S. Riquelme, J. Geng, F. Bravo, J. Carlos Baez, H. Parra, S. Barrientos, P. Fang, Y. Bock, M. Bevis, D. J. Caccamise, C. Vigny, M. Moreno and R. Smalley Jr. (2016), Local Tsunami Warnings: Perspectives from Recent Large Events, Geophys. Res. Lett., 43, 1109–1117, doi:10.1002/2015GL067100.
- Melgar, D. and Y. Bock (2015), Kinematic earthquake source inversion and tsunami inundation prediction with regional geophysical data, J. Geophys. Res. Solid Earth, 120, doi:10.1002/2014JB011832.
- Melgar, D. and Y. Bock (2013), Near-Field Tsunami Models with Rapid Earthquake Source Inversions from Land and Ocean Based Observations: The Potential for Forecast and Warning, J. Geophys. Res., 118. doi:10.1102/2013JB010506.
External References
- Atwater, Brian F, and Eileen Hemphill-Haley. 1997. "Recurrence intervals for great earthquakes of the past 3,500 years at northeastern Willapa Bay, Washington." In.: USGPO; Information Services [distributor].
- Hayashi, Toshihiko. 2012. 'Japan's Post‐Disaster Economic Reconstruction: From Kobe to Tohoku', Asian Economic Journal, 26: 189-210.
- Kido, M, Y Osada, H Fujimoto, R Hino, and Y Ito. 2011. 'Trench‐normal variation in observed seafloor displacements associated with the 2011 Tohoku‐Oki earthquake', Geophysical Research Letters, 38.
- Mimura, Nobuo, Kazuya Yasuhara, Seiki Kawagoe, Hiromune Yokoki, and So Kazama. 2011. 'Damage from the Great East Japan Earthquake and Tsunami-a quick report', Mitigation and adaptation strategies for global change, 16: 803-18.
- Mochizuki, M, T Kanazawa, K Uehira, T Shimbo, K Shiomi, T Kunugi, S Aoi, T Matsumoto, S Sekiguchi, and N Yamamoto. 2016. "S-net project: Construction of large scale seafloor observatory network for tsunamis and earthquakes in Japan." In AGU Fall Meeting Abstracts.
- Mori, Nobuhito, Tomoyuki Takahashi, and The Tohoku Earthquake Tsunami Joint Survey Group. 2012. 'Nationwide post event survey and analysis of the 2011 Tohoku earthquake tsunami', Coastal Engineering Journal, 54.
- Paris, Raphael, Franck Lavigne, Patrick Wassmer, and Junun Sartohadi. 2007. 'Coastal sedimentation associated with the december 26, 2004 tsunami in lhok nga, west banda aceh (sumatra, indonesia)', Marine Geology, 238: 93-106.
- Satake, Kenji, Kunihiko Shimazaki, Yoshinobu Tsuji, and Kazue Ueda. 1996. 'Time and size of a giant earthquake in Cascadia inferred from Japanese tsunami records of January 1700', Nature, 379: 246-49.
- Sato, Mariko, Tadashi Ishikawa, Naoto Ujihara, Shigeru Yoshida, Masayuki Fujita, Masashi Mochizuki, and Akira Asada. 2011. 'Displacement above the hypocenter of the 2011 Tohoku-Oki earthquake', Science, 332: 1395-95.
- Yun, Nam-Yi, and Masanori Hamada. 2014. 'Evacuation behavior and fatality rate of residents during the 2011 Great East Japan earthquake and tsunami', Earthquake Spectra, 31: 1237-65.